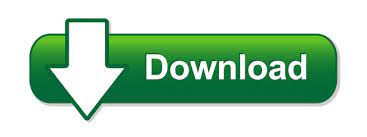
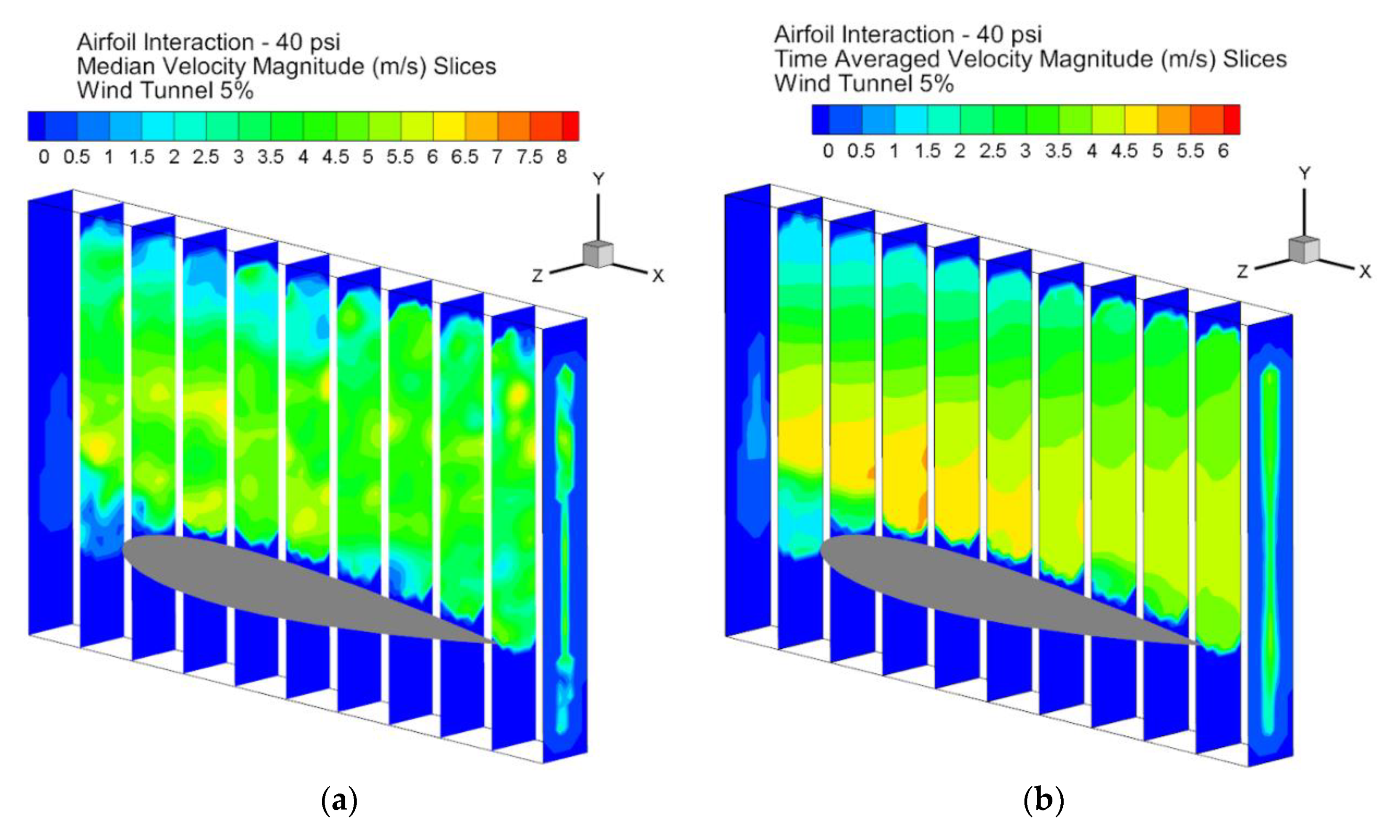

Therefore, the laminar to turbulent transition and the instability procedures significantly affect the unstable characteristics of LSBs and the mean flow topology. The separation and reattachment points are affected by these structures and they inherently cause the shape and size of the separated region to change. LSBs have an unsteady separated shear layer because of the small disturbances and these disturbances cause the vorticial structures to grow rapidly. But, if the LSB causes the local and limited impacts on the pressure distribution, it is ‘short’. If the pressure field is drastically affected by strong downstream and upstream impacts, it is ‘long’.

Assessment of effects of either ‘short’ or ‘long’ bubbles on the pressure distribution can be the best option and intuitive way. Aerodynamic researchers recommended a few parameters so that LSBs could be classified whether they are ‘short’ or ‘long’. Regarding a better understanding of LSBs’ topology, they can be categorized as ‘short’ and ‘long’ bubbles. Besides this, it causes the increment of unsteadiness and noise, especially for wind turbine applications. LSBs can form in many aeronautical applications operating in low Re regime of less than 1 × 10 6 and angle of attack (AoA) of less than stall angle, such as high latitude aircrafts, micro air vehicles (MAVs), multielement airfoil configurations, unmanned aerial vehicles (UAVs), wind turbine, and low-pressure turbine blades.Īs stated above, the aerodynamic efficiency can be severely decreased by LSBs by reducing the lift and increasing the drag forces. The region between separation and reattachment points is called as laminar separation bubble (LSB), which negatively affects the aerodynamic performance. Transition phenomenon in the separated region is caused by the separated shear layer, and then the turbulent reattachment starts to occur because of energized vorticial structures.

A laminar boundary layer can separate from the solid surface when the adverse pressure gradients (APGs) play a preponderant role. These flow phenomena commonly occur at low Reynolds numbers (Re) at which laminar flow is dominant. It is predicted that the fuel cost of a commercial aircraft could be saved to 8% if the transition phenomenon over its wing could be delayed to 50%. In particular, new types of these methods presented for the first time by the chapter’s authors have enormously influenced the progress of separation and LSB, resulting in postponing of the stall and enhancing the aerodynamic performance of wind turbine applications.īoundary layer transition and separation phenomena have been researchable topics for over 100 years, but there are still many open essential issues and practical challenges containing their controls. The control of the LSB with new concept pre-stall control techniques provides lift increment and drag reduction by utilizing significantly less matter consumption and low energy. Results indicate that passive flow control methods can dramatically increase the aerodynamic performance of the aforementioned vehicles by controlling the LSB occurring in the pre-stall region. In addition to the traditional methods, a new concept of the pre-stall controller by means of roughness material, flexibility and partial flexibility is highlighted with experimental and numerical results. This chapter presents a detailed review of traditional passive control techniques for flight vehicle applications operating at low Reynolds numbers. Thus, these vehicles require flow control techniques to reduce flow phenomena such as boundary layer separation or laminar separation bubble (LSB) affecting aerodynamic performance negatively. Prevailing utilization of airfoils in the design of micro air vehicles and wind turbines causes to gain attention in terms of determination of flow characterization on these flight vehicles operating at low Reynolds numbers.
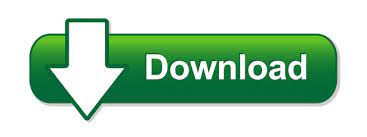